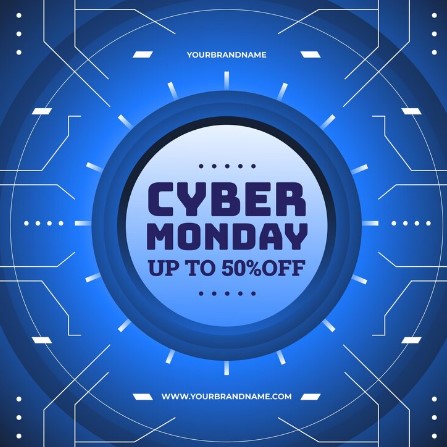
In the realm of technological innovation, advanced materials science stands at the forefront, promising groundbreaking solutions to some of the most pressing challenges facing humanity. From enhancing energy efficiency and enabling sustainable manufacturing to revolutionizing healthcare and transportation, advanced materials offer a myriad of opportunities for innovation and progress. This comprehensive exploration delves into the key principles, cutting-edge advancements, and real-world applications of advanced materials science, illuminating the path towards a more sustainable, efficient, and resilient future.
Understanding Advanced Materials Science
Advanced materials science is a multidisciplinary field that encompasses the design, synthesis, characterization, and application of novel materials with tailored properties and functionalities. Unlike traditional materials, which are limited in their capabilities and performance, advanced materials exhibit extraordinary properties such as strength, durability, conductivity, and biocompatibility, making them ideal candidates for a wide range of applications across various industries. By leveraging principles from physics, chemistry, engineering, and nanotechnology, advanced materials scientists strive to develop materials with superior performance, enhanced functionality, and reduced environmental impact.
Key Principles of Advanced Materials Science
Several key principles underpin the field of advanced materials science:
1.Structure-Property Relationships: Advanced materials scientists seek to understand the relationship between the atomic and molecular structure of materials and their macroscopic properties and behaviors. By manipulating the composition, morphology, and processing conditions of materials, scientists can tailor their properties to meet specific application requirements, such as mechanical strength, electrical conductivity, or optical transparency.
2.Nanotechnology and Nanomaterials: Nanotechnology plays a central role in advanced materials science, enabling the design and fabrication of materials at the nanoscale level. Nanomaterials, such as nanoparticles, nanotubes, and nanocomposites, exhibit unique properties and behaviors due to their small size and high surface-to-volume ratio, making them suitable for applications ranging from drug delivery and electronics to environmental remediation and energy storage.
3.Biomimicry and Bioinspiration: Biomimicry involves drawing inspiration from nature’s design principles and biological systems to develop advanced materials with enhanced performance and functionality. By mimicking the hierarchical structures, chemical compositions, and functional properties found in natural materials, scientists can create bioinspired materials that exhibit remarkable strength, flexibility, and self-healing capabilities.
4.Sustainable Materials Design: Sustainable materials design focuses on developing materials that minimize environmental impact throughout their lifecycle, from raw material extraction and manufacturing to use and disposal. By incorporating renewable resources, recycling principles, and eco-friendly production processes, advanced materials scientists can create sustainable alternatives to conventional materials, reducing resource depletion, pollution, and waste generation.
Innovations in Advanced Materials Science
The field of advanced materials science is characterized by continuous innovation and discovery, driven by advances in scientific knowledge, technological capabilities, and interdisciplinary collaboration. Some of the most groundbreaking innovations in advanced materials science include:
1.Graphene and Two-Dimensional Materials: Graphene, a two-dimensional carbon material with exceptional strength, conductivity, and flexibility, has garnered significant attention for its potential applications in electronics, energy storage, and biomedical devices. Researchers are exploring novel synthesis methods and functionalization strategies to harness the unique properties of graphene and other two-dimensional materials for diverse applications.
2.Metamaterials and Photonic Crystals: Metamaterials are artificial materials engineered to exhibit properties not found in naturally occurring materials, such as negative refractive index and invisibility cloaking. Photonic crystals, which manipulate the flow of light at the nanoscale, hold promise for applications in optical communication, sensors, and photonic devices. Researchers are developing novel fabrication techniques and design strategies to create metamaterials and photonic crystals with tailored optical properties and functionalities.
3.Additive Manufacturing and 3D Printing: Additive manufacturing, or 3D printing, enables the rapid prototyping and fabrication of complex structures and components with precise geometries and customizable properties. From aerospace components and medical implants to architectural structures and consumer goods, additive manufacturing offers a versatile and cost-effective approach to producing advanced materials and products with reduced material waste and production time.
4.Smart and Functional Materials: Smart materials are materials that exhibit adaptive, responsive, or programmable properties in response to external stimuli, such as temperature, light, or mechanical stress. Shape memory alloys, piezoelectric materials, and electrochromic polymers are examples of smart materials that find applications in actuators, sensors, energy harvesters, and adaptive structures. Researchers are exploring novel materials and design concepts to create functional materials with enhanced responsiveness and tunability.
Real-World Applications and Impact
Advanced materials science has already made a profound impact across various sectors and industries, driving innovation, economic growth, and societal progress. From lightweight composites and high-performance ceramics to nanomaterial-based coatings and biodegradable polymers, advanced materials are enabling breakthroughs in fields such as aerospace, automotive, healthcare, energy, and environmental sustainability. By enhancing efficiency, durability, and performance while reducing environmental footprint and resource consumption, advanced materials science is paving the way for a more sustainable, efficient, and resilient future.
Challenges and Opportunities
Despite the significant progress made in advanced materials science, several challenges remain to be addressed, including scalability, cost-effectiveness, and safety concerns associated with the production and use of advanced materials. Additionally, issues related to intellectual property rights, regulatory frameworks, and ethical considerations must be carefully considered to ensure responsible innovation and equitable access to advanced materials technologies. Nonetheless, the potential of advanced materials science to drive positive change, spur economic development, and address global challenges is immense, offering unprecedented opportunities for collaboration, discovery, and impact.
Conclusion
In conclusion, advanced materials science represents a transformative force for innovation, sustainability, and progress in the 21st century. By pushing the boundaries of scientific knowledge, technological capabilities, and interdisciplinary collaboration, advanced materials scientists are unlocking the potential of next-generation materials to address pressing societal challenges and create a more sustainable, efficient, and resilient world. As we embrace the promise of advanced materials science, let us work together to harness its power for the benefit of humanity and the planet, ensuring a brighter future for generations to come.